Research Focus
.png?sfvrsn=c41c35f5_1/)
Quantum Software and Algorithms
FOCUS:
- Compiler & Intermediate Representations (IR): Develop scalable, memory-efficient IRs optimized for continuous-variable photonic platforms. Leverage advanced tools like ZX-calculus to streamline circuit manipulation, integrate error correction, and accommodate unique hardware constraints.
- Advanced Emulation Tools: Build high-performance tensor-network-based emulators that accurately model photonic quantum systems and beyond, including non-Markovian noise, crosstalk, and continuous-variable operations, to support realistic testing and validation of quantum hardware virtualization.
- AI-Enhanced Optimization: Employ AI-driven techniques, such as transformer-based neural networks, to improve circuit compilation, error correction efficiency, and scalability, adapting to evolving hardware and resource limitations.
- Rigorous Validation & Benchmarking: Establish robust benchmarks and validation protocols to ensure firmware scalability, reliability, and compatibility with thousands of logical qubits on photonic quantum hardware in the future.
- Integration with Hardware Developments: Align closely with quantum hardware development in Q.InC, including control electronics and critical quantum components, ensuring a seamless, hardware-aware quantum firmware stack.
Quantum Photonic Components
FOCUS:
To develop critical device components for integrated quantum photonics platforms towards developing a fully integrated photonics quantum computer. This is divided into three areas:
- Thin-film lithium niobate platform
- Nanophotonic devices for generating and controlling quantum light
- Integrated photodetectors
Quantum Sensing and Metrology
The Quantum Sensing and Metrology (QSM) pillar focuses on harnessing quantum phenomena to achieve unprecedented sensitivity and precision in sensing application. We address key challenges in quantifying the quantum advantage, optimizing sensor performance, and identifying practical use cases where quantum sensors outperform classical alternatives. Our research spans the full spectrum of quantum sensing, including atomic vapor cell sensors, levitation sensors, and quantum imaging & spectroscopy.
Quantum Optical Resources and Quantum Metrology
Advances in quantum information have revolutionized sensing technologies, enabling breakthroughs like the quantum Fisher information for quantifying the ultimate limits of quantum resources. Building on these theoretical advances, we explore practical approaches to preserve quantum advantages in real-world, noisy environments and investigate novel concepts such as distributed and error-mitigated quantum sensing. Optical technologies, the backbone of many sensors, benefit from quantum optical states like entangled photons and squeezed light, which enhance sensitivity and precision. By developing scalable methods to generate these quantum resources and integrating advanced optical readout components, we aim to unlock the full potential of quantum sensing for diverse real-world applications.
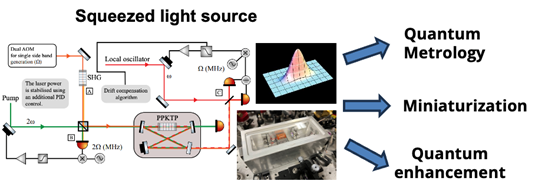
Atomic Vapor Sensors
Atomic vapor-based sensing technology is at the heart of numerous precision sensing and metrology application ranging from magnetometry to time-keeping and inertial navigation. We are developing next-generation atomic magnetometers with ultra-sensitive magnetic field detection, without the need for cryogenic cooling required by conventional SQUIDs. This cost-effective and portable technology can revolutionize applications such as brain and heart imaging, mineral exploration, and geomagnetic surveys. It also enables compact and affordable nuclear magnetic resonance (NMR) and MRI systems by removing the need for large, expensive magnets. Additionally, our magnetometers can probe nuclear quadrupole resonance (NQR), offering new possibilities in pharmaceutical quality control and explosives or narcotics detection.
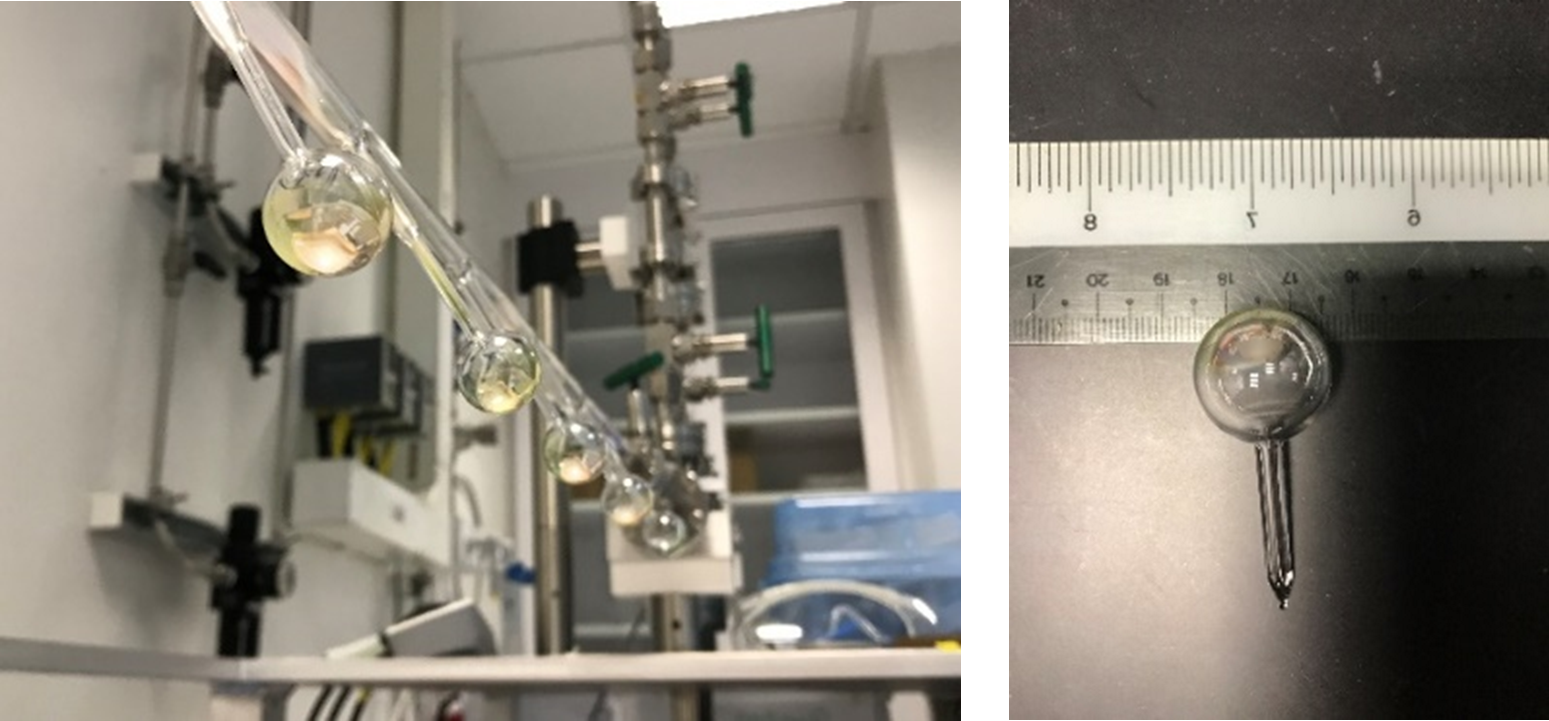
Coherent Levitation Sensor
Optomechanical systems combine optical fields with mechanical motion to build some of the most precise sensors in existence. The most famous example is the Laser Interferometer Gravitational-Wave Observatory (LIGO), a four-kilometre-long optomechanical system, which measures spacetime distortions 10,000 times smaller than a proton. Recent years have seen the explosive growth of levitated optomechanics, which uses optical or electromagnetic fields to suspend systems without physical connection to the environment. The resulting isolation allows us to approach the quantum ground state even at room temperature. However, existing methods such as optical tweezers are limited to micro particles. They also involve incoherent interactions where light scatters off the particle, which excludes the possibility of optical quantum control and measurement.
At Q.InC we are pioneering coherent levitation of large masses, to enable quantum control and readout of milligram-scale sensors. These could act as ultra-precise sensors of gravity, acceleration, or electromagnetic fields. They would also allow us to explore quantum and gravitational effects in unprecedented regimes. Our platforms are: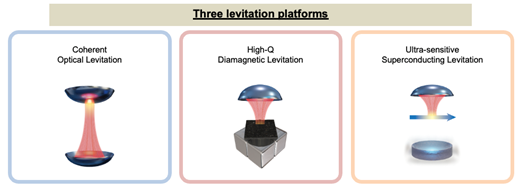
Quantum Imaging & Spectroscopy
We are advancing infrared metrology and imaging technologies using quantum principles such as induced coherence without induced emission. This approach enables the detection of infrared material information through visible light, leveraging low-cost, widely available light sources and detectors for significant cost savings and flexibility. In addition, combining advanced hyperspectral microscopy with quantum light sources could open new regime of imaging and spectroscopy technologies, achieving superior signal-to-noise ratios and biologically safe operation. These innovations pave the way for transformative breakthroughs in quantum material characterization, biosensing, biomedical imaging, and other cutting-edge applications.
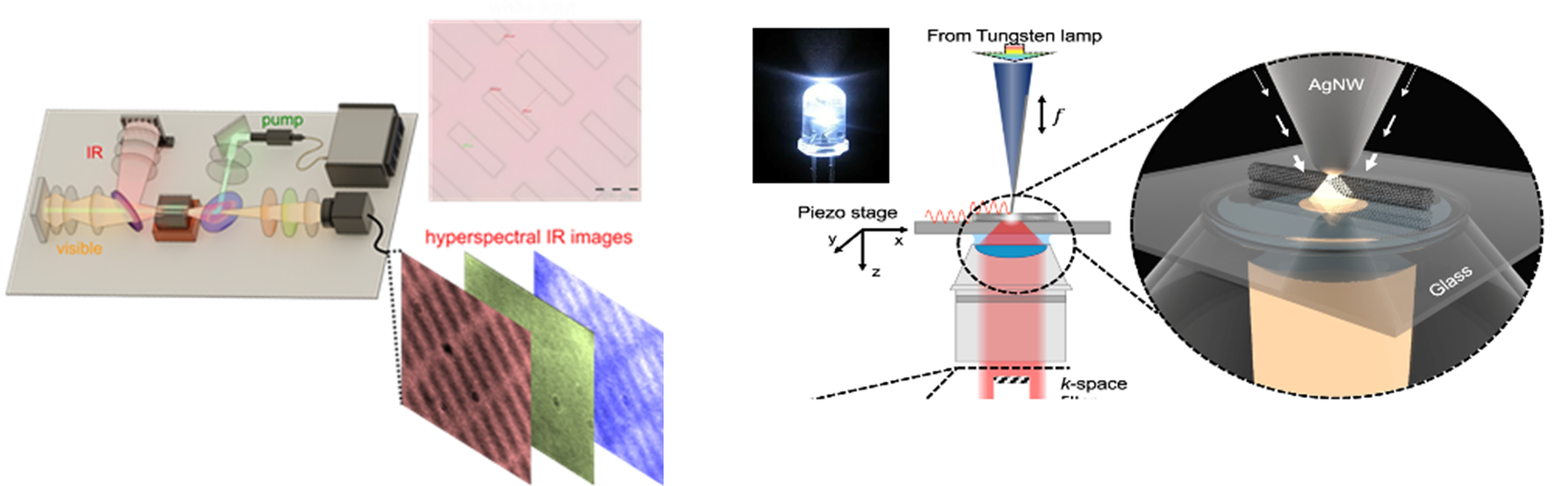
Quantum Optical Resources and Quantum Metrology
Advances in quantum information have revolutionized sensing technologies, enabling breakthroughs like the quantum Fisher information for quantifying the ultimate limits of quantum resources. Building on these theoretical advances, we explore practical approaches to preserve quantum advantages in real-world, noisy environments and investigate novel concepts such as distributed and error-mitigated quantum sensing. Optical technologies, the backbone of many sensors, benefit from quantum optical states like entangled photons and squeezed light, which enhance sensitivity and precision. By developing scalable methods to generate these quantum resources and integrating advanced optical readout components, we aim to unlock the full potential of quantum sensing for diverse real-world applications.
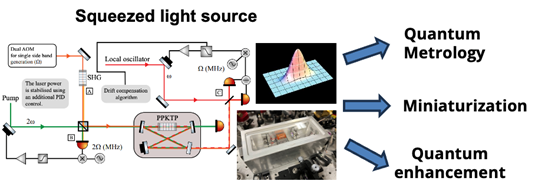
Atomic Vapor Sensors
Atomic vapor-based sensing technology is at the heart of numerous precision sensing and metrology application ranging from magnetometry to time-keeping and inertial navigation. We are developing next-generation atomic magnetometers with ultra-sensitive magnetic field detection, without the need for cryogenic cooling required by conventional SQUIDs. This cost-effective and portable technology can revolutionize applications such as brain and heart imaging, mineral exploration, and geomagnetic surveys. It also enables compact and affordable nuclear magnetic resonance (NMR) and MRI systems by removing the need for large, expensive magnets. Additionally, our magnetometers can probe nuclear quadrupole resonance (NQR), offering new possibilities in pharmaceutical quality control and explosives or narcotics detection.
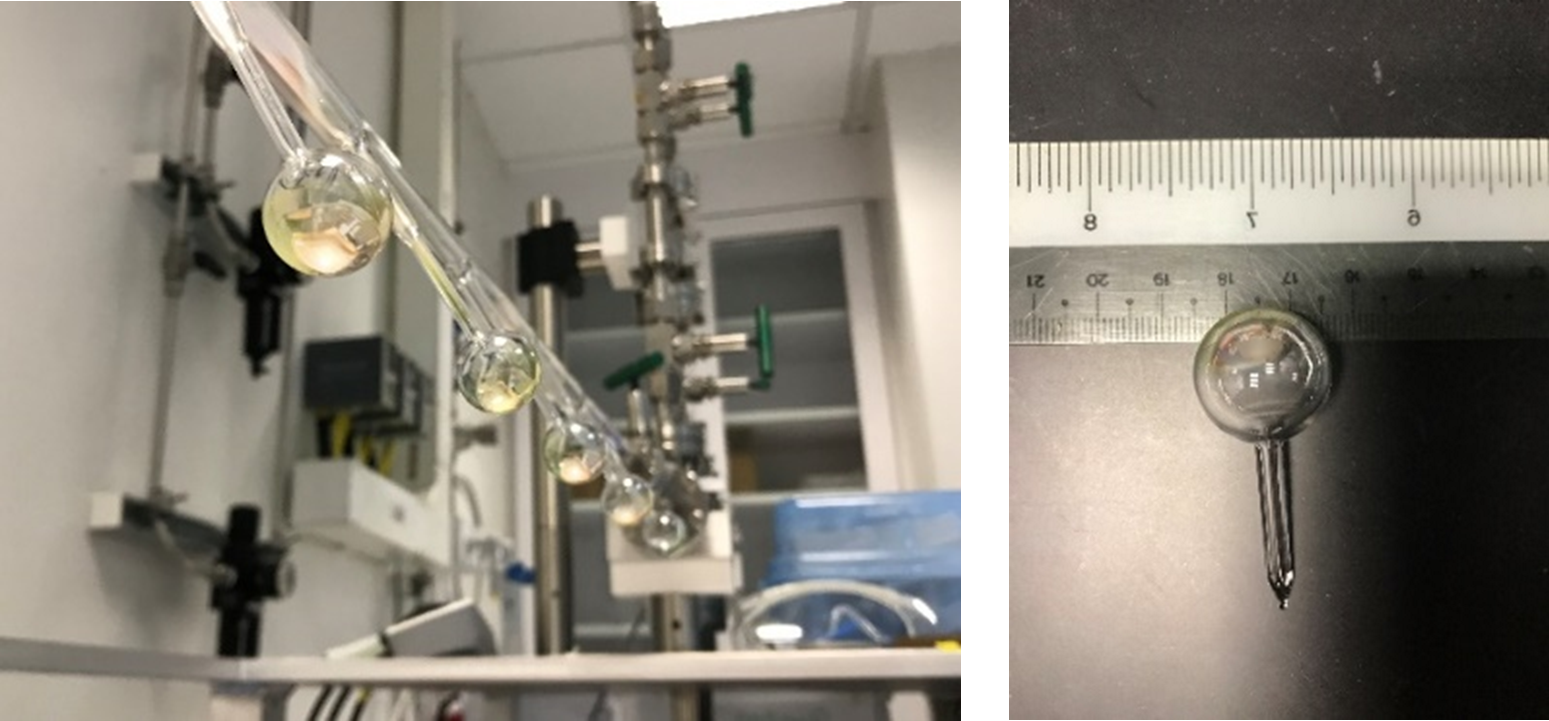
In-house capability to fabricate custom atomic vapor cells
Coherent Levitation Sensor
Optomechanical systems combine optical fields with mechanical motion to build some of the most precise sensors in existence. The most famous example is the Laser Interferometer Gravitational-Wave Observatory (LIGO), a four-kilometre-long optomechanical system, which measures spacetime distortions 10,000 times smaller than a proton. Recent years have seen the explosive growth of levitated optomechanics, which uses optical or electromagnetic fields to suspend systems without physical connection to the environment. The resulting isolation allows us to approach the quantum ground state even at room temperature. However, existing methods such as optical tweezers are limited to micro particles. They also involve incoherent interactions where light scatters off the particle, which excludes the possibility of optical quantum control and measurement.
At Q.InC we are pioneering coherent levitation of large masses, to enable quantum control and readout of milligram-scale sensors. These could act as ultra-precise sensors of gravity, acceleration, or electromagnetic fields. They would also allow us to explore quantum and gravitational effects in unprecedented regimes. Our platforms are:
- Optical: Photons carry momentum, so when they reflect from a mirror they impart radiation pressure force. We are using this to lift a milligram mass, which will levitate on a dynamically tunable 'optical spring'.
- Diamagnetic: Graphite can levitate indefinitely with zero power consumption. We are developing nanofabricated graphite composites which exhibit extremely low levels of dissipation, providing a pathway to centimetre-sized quantum sensors.
- Superconducting: At cryogenic temperatures thermal noise is greatly suppressed, allowing quantum effects such as superconductivity to come to the fore. We are building a micrometre ferromagnetic needle where the quantum spin of the individual atoms combines into a large 'macro-spin'. This would be an extremely sensitive magnetometer, and allow us to observe the effects of quantum spin on macroscopic scales.
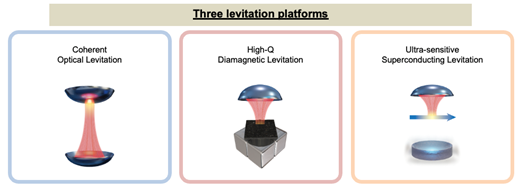
Quantum Imaging & Spectroscopy
We are advancing infrared metrology and imaging technologies using quantum principles such as induced coherence without induced emission. This approach enables the detection of infrared material information through visible light, leveraging low-cost, widely available light sources and detectors for significant cost savings and flexibility. In addition, combining advanced hyperspectral microscopy with quantum light sources could open new regime of imaging and spectroscopy technologies, achieving superior signal-to-noise ratios and biologically safe operation. These innovations pave the way for transformative breakthroughs in quantum material characterization, biosensing, biomedical imaging, and other cutting-edge applications.
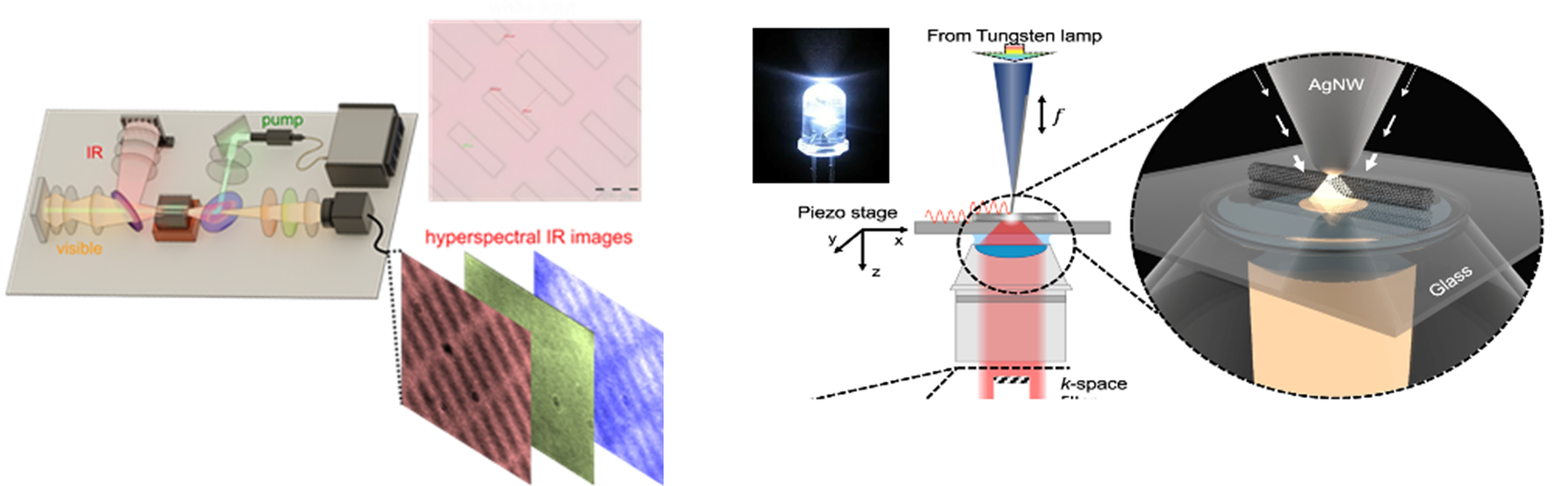
Quantum Materials and Devices
Quantum Materials and Applications
FOCUS:
- Advanced Packaging: Development of 2.5D/3D heterogeneous integration techniques, including silicon interposers with superconducting interconnects, through-silicon vias (TSVs) for efficient, scalable quantum device interconnections at cryogenic temperatures.
- Cryogenic CMOS: Design and characterization of cryogenic CMOS circuits for qubit control and readout, focusing on low-power, low-noise logic and optimizing performance for ultra-low temperature environments, as well as implementing quantum error correction (ECC) strategies.
- Silicon Photonics: Development of silicon photonic devices for photon generation and sensing at cryogenic temperatures, including on-chip light sources, single-photon detectors, and integrated photodetectors for quantum optics and sensing applications in quantum computing.
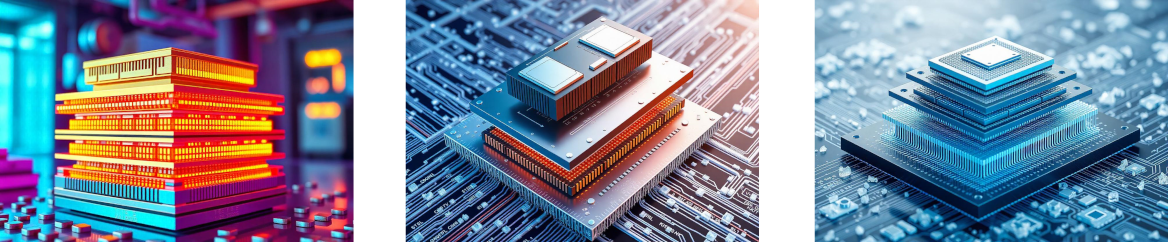
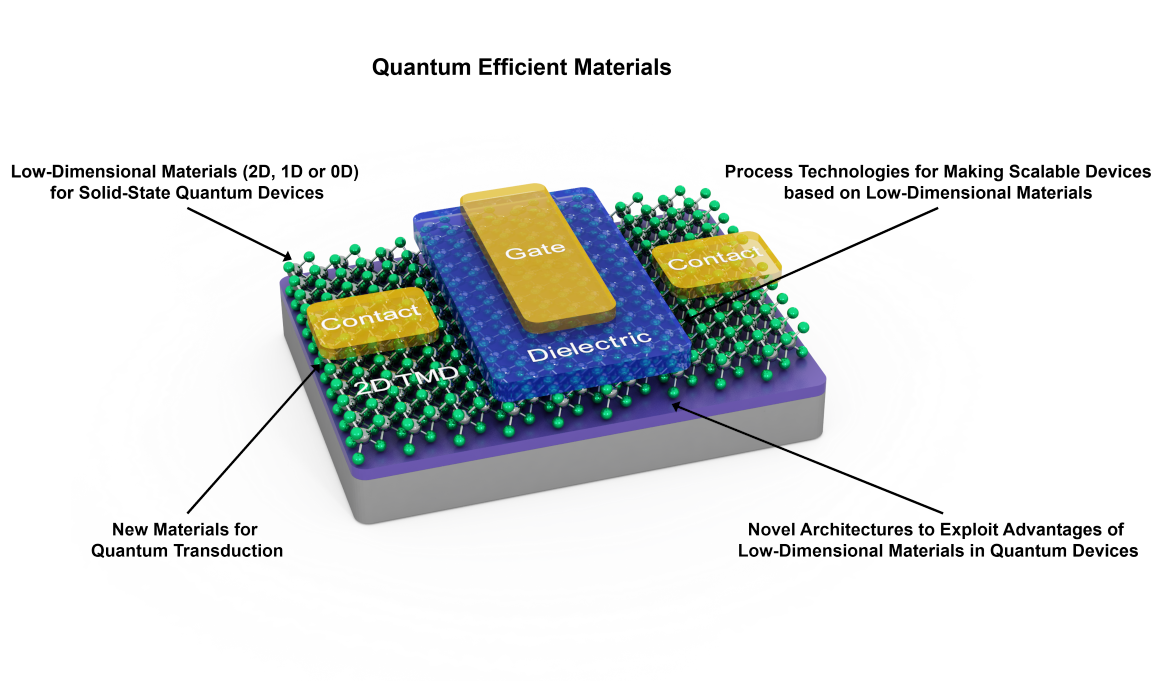
A*STAR celebrates International Women's Day
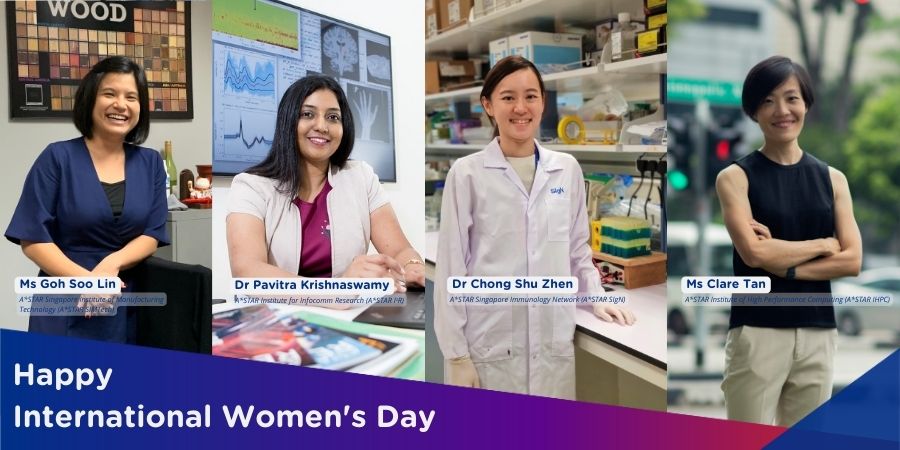
From groundbreaking discoveries to cutting-edge research, our researchers are empowering the next generation of female science, technology, engineering and mathematics (STEM) leaders.
Get inspired by our #WomeninSTEM