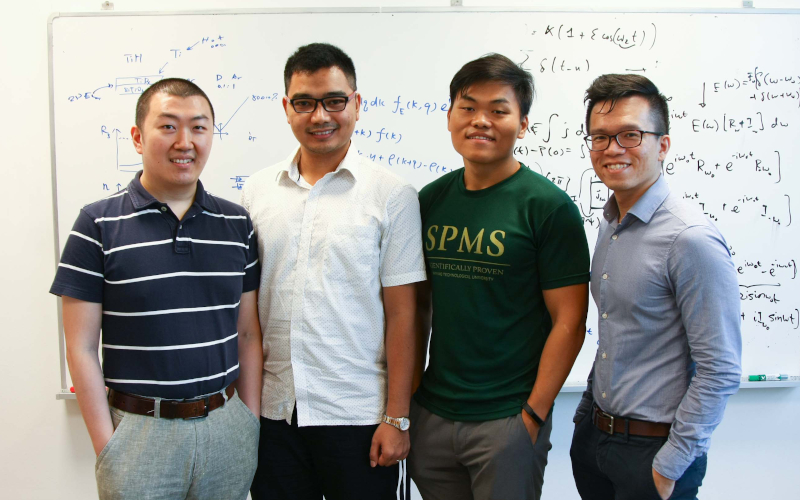
(From right) Dr Justin Song and his team (Mr Ruo Cheng Huang, Dr Eddwi Hesky Hasdeo, Dr Likun Shi).
Over the years, the dizzying pace of scientific research has brought many technologies out of the realm of science fiction and into reality. These days, we barely bat an eye at the prospect of driverless cars or cutting and pasting DNA. Still, there’s one field with discoveries that routinely sound straight out of science fiction—quantum physics.
What, then, makes quantum physics seem so bizarre? For starters, many of its basic principles appear to defy our everyday instincts of how the world should work. Consider quantum entanglement, where quantum particles can become ‘entangled’ such that any action on one of the particles instantly affects the other, even at great distances.
But by exploiting these principles, researchers can unlock a vast world of possibilities, with far-reaching implications beyond the quantum realm. Justin Song, a Senior Scientist at A*STAR’s Institute of High Performance Computing (IHPC) and Nanyang Assistant Professor at Nanyang Technological University, is one such researcher exploring how quantum effects manifest in different materials.
Specifically, Song’s theoretical work tackles the use of light in altering the properties of materials, like potentially turning a non-magnetic metal into a magnet. For unveiling the novel properties of quantum materials, Song was conferred the prestigious Young Scientist Award in 2017. In this conversation with A*STAR Research, Song walks us through the weird, wonderful world of quantum materials and shares his take on the wider relevance of his research.
1. How did you become interested in your field of research?
I think it was largely by accident. While I've always been interested in science in general, I would say I stumbled into my area—condensed matter physics and quantum materials—by chance.
2. Tell us about the key problem you are trying to solve with your research.
It is a little hard to pinpoint a specific key problem that we’re trying to solve since we’re actively pushing on multiple fronts. But broadly speaking, we're trying to come up with new ‘out-of-equilibrium’ strategies for designing quantum material behavior. Typically, quantum devices work at or close to thermodynamic equilibrium, where energy is uniformly distributed among all components in a system. Many thermodynamic factors restrict material performance and behavior at equilibrium, but these constraints quickly fall away when materials are pushed far out-of-equilibrium. Therefore, one of our research directions is formulating new means for manipulating energy, charge or spin when a material is excited out-of-equilibrium.
A concrete example of the importance of investigating systems that are out-of-equilibrium involves spintronics devices. In spintronics, an electron’s spin is used to carry information, instead of its electrical charge. Compared to conventional electronics, spintronics is a promising alternative that enables the faster processing of information despite using less energy.
To create a spintronics device, spin currents must be injected into semiconductor materials from magnetized metal cheaply and efficiently. However, transferring these spin currents is a challenging process that requires conditions close to equilibrium. In a recent study published in Nature Physics, we showed that heating a cobalt ferromagnet using laser pulses generates spin-carrying electrons unevenly distributed in energy—in other words, ‘out-of-equilibrium’—that then selectively diffuse into the semiconductor. So far, our technique has proven to be much simpler and more efficient compared to other methods.
3. What are some recent developments in your field that you intend to build upon?
There are lots of developments that we’re building on in our field. One particularly striking recent finding is that of ‘twistronics,' the study of emergent behavior (e.g., superconductivity) that occurs when two seemingly ordinary layers of graphene are twisted together at a special ‘magic’ angle. This means that while electrons in a single layer behave like a normal metal, the electrons in twisted graphene bilayers move in a way that exhibits zero resistance. In other words, electrons can travel without dissipating energy, turning the bilayer into a superconductor with a simple twist. This very unusual behavior is not understood, and we intend to find out what components go into supporting this behavior.
4. Could you please describe one of the most exciting projects you are working on right now?
One thing we’re most excited about now concerns sustaining topological, or deformed, states out-of-equilibrium. There is a class of materials called topological materials with useful properties like one-way electronic channels that possess very low resistance. These materials are hard to synthesize, often require exotic configurations, and/or manifest at super low temperatures.
One idea that has been gaining traction recently is using laser light to warp the structure of a material so that it exhibits topological properties (e.g., one-way electronic channels) while the light is turned on. These are called Floquet topological insulators. However, Floquet topological insulator states require tremendous light power to induce and they vanish rapidly once the light is turned off. We are currently working on a protocol that can ‘freeze’ some of the properties of these topological states for long periods of time even when the light is off.
5. What are some of the implications of your research? Who will benefit from the findings?
It is very difficult to predict who will directly benefit from our findings since our work is largely theoretical. What we mainly do is chart out the possibilities and strategies that engineers and experimentalists can undertake to realize completely new behavior. We’re like cartographers mapping out the routes forward. I anticipate that our work will benefit the scientists and quantum engineers looking to building new types of quantum devices and sensors that rely on the delicate quantum properties that can be sustained in materials.